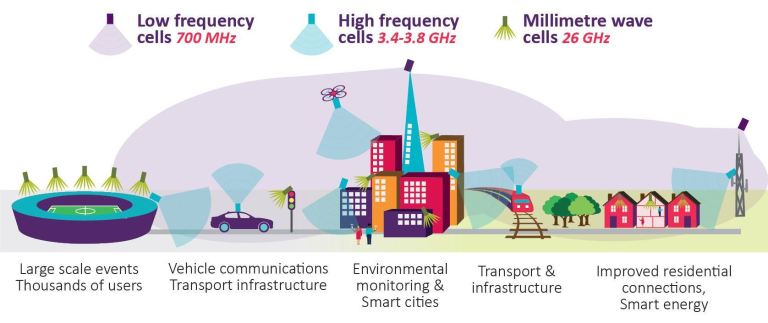
Project Overview
The wireless communication network has been a great success. Benefiting from the simple architecture of the Internet Protocol, people at any place can access any content available on the internet, regarless of texts, audios, even videos. The utility of high speed data connection has expanded rapidly from the original purpose of simple computer messaging to everything in our everyday lives today, including healthcare, weather forecast, entertainment, and shopping. The exponential growths in the Internet traffic have continued impressively from one TB/month in 1990 to 10 million TB/month in 2014, and is projected to exceed 100 million TB/month by year 2020 [1].
While the increased traffic volume is remarkable on its own, the Internet applications have evolved from fixed desktop environments to cloud environments that emphasize ubiquity, mobility, and bandwidth. Future Internet applications are expected to demand even more ubiquity, mobility, and bandwidth through diverse platforms, in particular, rapidly expanding cloud data center infrastructures. How do we cope with such increasingly challenging demands and requirements in the future? Today’s wireless networks are typically limited to 1~100 Mb/s connectivity with poor end-to-end throughput, latency, and reliability [2]. While fiber optic networks provide 1~100 Tb/s capacity on each single-mode-fiber over thousands of kilometer distances, they are limited to wide area and metro networks, not readily accessible by mobile users.
On the other hand, there have been three new important developments [3]: (1) The future 5G network standard has set the goal of traffic throughput at least over 20Gbps, and several prototype demostrations have been reported[4]. (2) The new elastic optical networking (EON) technologies utilizing coherent optical communications are emerging as a viable future platform for wide area networking, and the EON technologies can seamlessly interface with mmWave communication technologies[5, 6].
- (3) Silicon photonics have become commercially available from a number of foundries to offer low-cost RF-photonic and coherent optical integrated circuits. Leveraging such new developments, can we bring > 100 Gb/s to mobile users with high quality of service end-to-end? Is it possible to make such networks ubiquitous and inexpensive for everyone? Can such networks be easily managed and controlled? Without doubt, a clean-slate and comprehensive investigation into new network architectures, system concepts, and component technologies is necessary to address these questions.
The proposed project pursues the first step to this goal by investigating elastic RF-optical networking (ERON) systems and technologies that bring mobility, ubiquity, and high bandwidth together with high quality of service.
Current Research Activities
1. Optical Signal Processing: One promising goal of ERON is to utilize the energy and cost efficient photonic technology to implement mmWave generation and transmission. The power loss and design difficulties of RF electronics surge as the frequency increasing from radio wave (MHz to several GHz) to mmWave (over 25GHz). Under ERON architecture, the mmWave generation and signal transmission are carried by lightwave, which is inherently low loss. Our interests focus on optical signal processing to replace expensive and energy consuming mmWave signal processing, photonic-integrated circuit for compact, low loss, and scalable implementations, etc.
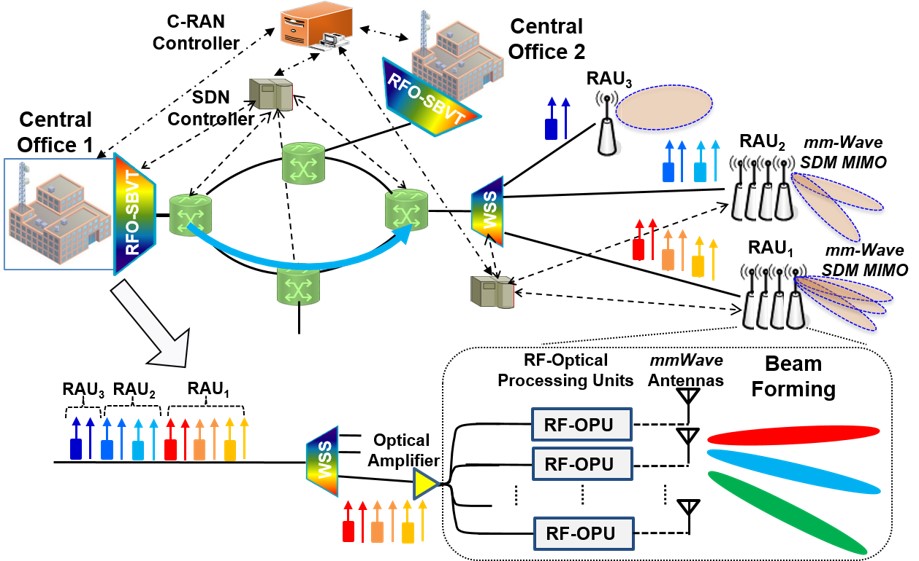
Figure 1. Illustration of an ERON architecture, integrating photonic signal processing with mmWave wireless beamforming signal transmission. RAU: remote antenna unit; MIMO-SDM: multi-input multi-output spatial division multiplexing; RFO-SBVT: RF-optical sliceable bandwidth variable transponder.
2. Coding and DSP algorithm for spatial-spectral photonic-wireless communication: A layered hybrid beamforming structure can compensate large propagation pathloss through beamforming and achieve high spectral efficiency through spatial multiplexing. By using relatively simple outer layer RF beamforming design, we can better utilize the directional propagation of mmWave signals and alleviate inner layer base-band precoding. With modelling of photonic components and precoding design, we aim to improve spectrum efficiency and overall system capacity.
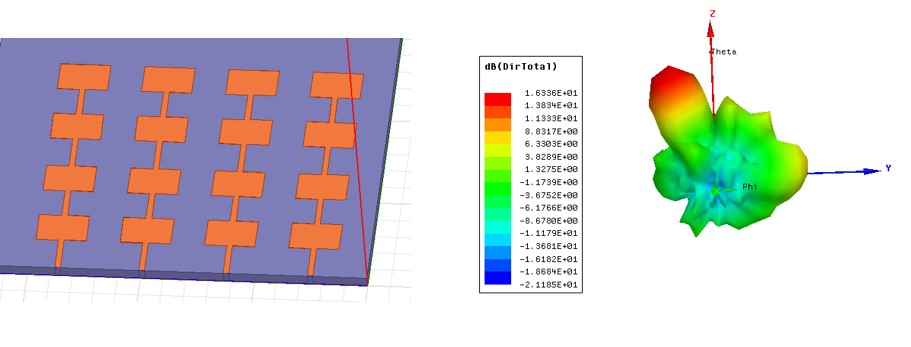
Figure 2. Block diagram for MIMO-OFDM signal at the transmitter and receiver.
3. Phase array antenna design: One of the ERON’s goals is to demonstrate high capacity (over 20Gbps) wireless throughput on an end-to-end wireless communication system, and design high directivity phased array antenna is a crucial component. Our interests in phased antenna range from proto-type PCB patch antenna array to integrated antenna on chips.
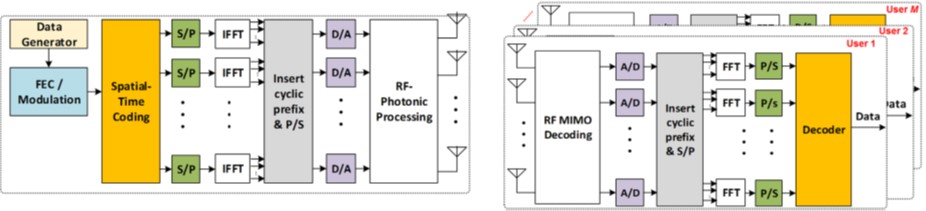
Figure 3. Design of a 4-by-4 PCB patch phased antenna array and simulated far-field beam pattern.
4. Control layer and management layer design with Software Defined Network: We investigate network level RMSA management algorithm and control layer implementation. We incorporate machine learning, linear programming, and other network modelling method with photonic-wireless heterogeneous networking control/management plane, and optimize network resources and resilience to high efficiency and strong robustness. Figure 4 shows our initial ERON demonstration of a reconfigurable photonic-wireless network [7].
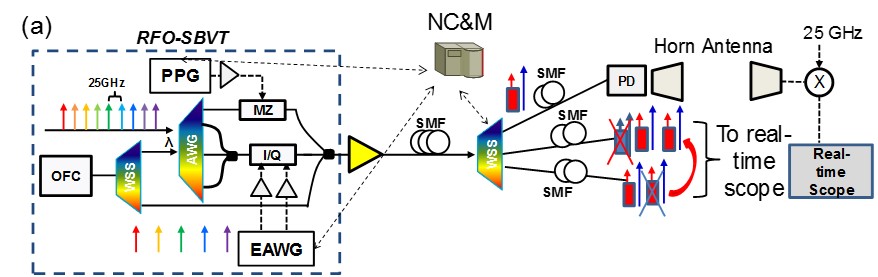
Figure 4. Demonstration of network reconfigurability of the ERON system. (a) ERON experiment testbed. WSS: wavelength selective switch; PPG: pulse pattern generator; EAWG: electrical arbitrary waveform generator; BERT: bit error tester; PD: photodetector; MZ: Mach Zehnder modulator; I/Q: phase/quadrature modulator; AWG: arrayed waveguide grating; OFC: optical frequency comb; WSS: wavelength selective switch. (b) OOK eye diagram and BER value after free-space transmission. (c) optical spectrum of the channel switching on link to RAU2. (d) BER measurements for RoF signals going to RAU2 and RAU3.
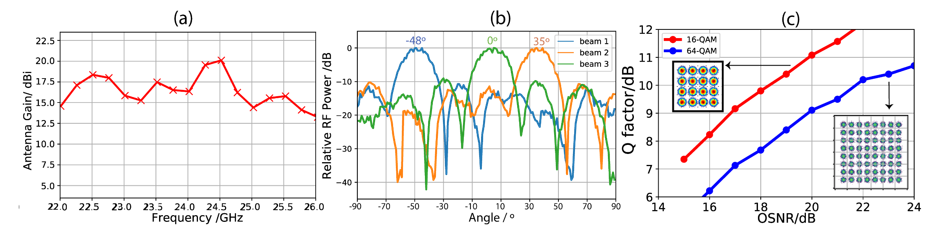
Figure 5. (a) Measured patch array antenna beampattern. (b) Measured beam steering results. (c) Experimental front-end transmission Q-factor vs OSNR result for 64-QAM and 16-QAM signal respectively.